Spotlight on: Synthetic biology
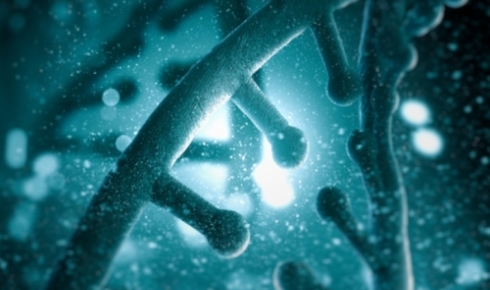
The Biologist Vol 61(6) p30-31
Synthetic biology is the engineering of biological systems to create useful products or devices. It has the potential to create new life forms with extraordinary properties that exist purely to meet human needs.
Why is it important?
Synthetic biology is one of the most exciting and fast-growing subdisciplines of the life sciences. Last year the Government identified it as one of the eight great technologies of the future and committed £40m to developing three new synthetic biology research centres in the UK. This reflects a global trend for massive investment in this type of research in recent years.
As well as splicing in genes from other organisms, novel sections of DNA can now be synthesised cheaply from scratch and inserted into organisms, creating experimental cells with new functions and features not seen before in nature. Synthetic biologists choose a 'chassis' organism and then add features such as genes, the promoters that activate them and the systems they drive.
There are many parallels with open source software programmers, but rather than sharing code, scientists share DNA sequences that code for a certain feature. Open registries of synthetic biology 'parts' have helped drive rapid innovation.
How do I get into it?
After the potential of synthetic biology was recognised around a decade ago, governments and global institutions set up an abundance of synthetic biology initiatives, networks and research centres. A huge amount of funding is available for projects with a synthetic biology slant and a range of competitions, such as iGEM, encourage innovative synthetic biology projects from teams in schools, universities and industry.
Thanks to cheap and commercially available toolkits, synthetic biology is also popular with the growing number of hobbyists known as 'biohackers' who use borrowed, homemade or unwanted lab equipment to conduct their experiments.
For those who would like to take a more traditional route, courses in synthetic biology tend to be at postgraduate level, most as one-year master's degrees. Imperial College London now offers a synthetic biology final year option as part of undergraduate degrees in biology, biochemistry or bioengineering, and some US universities such as Berkeley allow a synthetic biology 'concentration' (specialisation) during certain bioscience degrees.
What careers are available?
The field offers many opportunities for early career scientists. Practical applications of the technology include the production of biofuels, vaccines and antibodies, industrial enzymes and chemicals, and biomaterials and agriculture, and there are placements in industry to reflect each of these areas. However, the majority of careers remain in academic research as researchers continue to refine techniques and explore the possibilities of this new field.
Where can I get more information?
iGEM advances synthetic biology through a large global competition for schools and universities.
www.iGEM.org
The BioBricks Foundation runs an annual international synthetic biology conference and provides resources for researchers.
www.biobricks.org
Research Councils UK's Roadmap sets out a vision for the future of synthetic biology in the UK. www.rcuk.ac.uk/publications/reports/syntheticbiologyroadmap
The Biotechnology and Biological Sciences Research Council and the Engineering and Physical Sciences Research Council are both large funders of synthetic biology research and have more resources on their websites.
www.bbsrc.ac.uk
www.epsrc.ac.uk
At a glance
Name: Dr Jim Haseloff
Profession: Reader in synthetic biology at the University of Cambridge, head of the Haseloff Lab, Cambridge
Qualifications: PhD (biochemistry), University of Adelaide; Research Fellow, MRC Laboratory of Molecular Biology; Research Fellow, Harvard University
Interests: Plant development and morphogenesis, precise monitoring of cell behaviour, engineering of tissues in plants
What do you do in your research lab?
There are two branches of our work. The first uses the Marchantia plant as a base organism for developing new plants. Our other work is looking at the way bacterial cells grow and interact – it sounds odd for a plant science lab, but bacterial cells offer the easiest access to cells' genomes and what happens as they grow.
How does your work with bacteria inform your work to develop new crops?
The genetic difference between the crop maize and its wild ancestor teosinte is minimal – the fact that maize is a useful crop is due to the way morphogenesis [the shape and relative size of parts of an organism] is controlled. If you can understand the changes that have been made over time to create maize from teosinte, you can potentially create very different versions of existing plants.
If you are trying to engineer morphogenesis in plants, it is the physical interactions between the cells that determine the eventual shape. You can't think about the shape of a group of cells without understanding the physics of how they divide and grow. When you scrape microbes on an agar plate, you'll often find they've formed perfect domes containing billions of cells within a few days. However, cells grow in one long string – it's a slight buckling of these cells as they divide that creates strange fractal patterns and an ordered structure. And that is what I am looking at.
How do you define synthetic biology?
I think it's a lot like how before the industrial revolution if you wanted to engineer something, you had to make it all from scratch. And then in around the 1830s, you began to see standardisation of parts so you didn't have to think about making absolutely everything, like the nuts and bolts. I think biology is in that place now. Simple standards underpin the processes of, say, manipulating or reprogramming cells.
What other types of 'standard parts' do you use?
Well, what's involved in making a living system? You have the chassis, then what do you want controlling that system – an entire genome, a control circuit made of DNA? Once you have that, you've got to use things to measure what's going on at the DNA, cellular, phenotype level. Then it's also often very important to have a computerised model of your system running alongside your experiment.
How did you end up getting into synthetic biology? Presumably it didn't exist as a field when you began your career?
No, it didn't exist in name or intent, I guess. I came into the field in the 1970s when it was molecular biology. Even then, pre-internet, it was still about tweaking and refining techniques. Except then you would fly round the world to a lab and make handwritten notes that you'd then photocopy and they would be like gold dust.
Your OpenPlant project recently received £12m funding. What is it like to work there?
The funding is split into two halves: here in Cambridge and the John Innes centre in Norwich. In Cambridge, it's more about the fundamental science; in Norwich, the focus is on trait development. It's a really exciting collaboration, putting people who have been working in fundamental science into a context of trait development.
What sort of traits are you looking at?
Creating high value pharmaceuticals and vaccines from plants, improved nitrogen fixing, and altered photosynthetic capabilities.
What other areas of synthetic biology are exciting for the future?
Metabolic engineering – creating molecules. The 1000 Molecule project creates biomaterials that can be used in different ways – for example, biological plastics. Light and creating bioluminescence is also something that really fascinates me.