The key to ‘the vault’
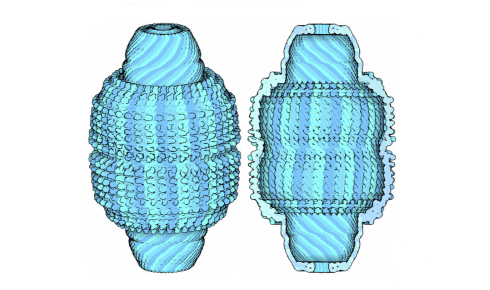
27th May 2024
Large, barrel-shaped particles known as vaults are found in huge numbers in the cells of many organisms, including humans. But what do they do? And why do so few biologists study them?
In 1986, UCLA researchers Nancy Kedersha and Leonard Rome were attempting to isolate cell vesicles, when they spotted a contaminating particle among the samples. Wondering if these oddly shaped particles might be related to the vesicles in some way, the team purified and characterised them. They were, it turned out, entirely unrelated to vesicles – made of protein and a small amount of RNA.
After a naming contest in the lab, the pair settled on a name based on the characteristic architecture of the particle’s wall, which was not unlike the vaulted ceiling of a cathedral. This is where the strange story of the mysterious cell organelles known as ‘vaults’ begins.
Abundant and everywhere
Vaults are one of the biggest naturally occurring particles in cells. At 70nm long, they are larger than a ribosome. And yet they are also simple – containing just three different proteins where a ribosome might contain a hundred.
The particles are present in a curious selection of organisms. Rome, now a distinguished professor of biological chemistry at UCLA, first saw vaults in rat liver cells, but has found them in most human cells too. They can be found across the eukaryotic kingdom – from ancient and simple eukaryotes such as protozoa to higher animals – but have been lost by large groups such as yeasts and plants. Annoyingly for Rome’s research, they are not present in classic model organisms such as nematodes or Drosophila either.
Where vaults are present, there are lots of them. Rome estimates that there are around 10,000 or so in a typical human cell. “Some quick math suggests you’ve got about 160 quadrillion vaults in you,” he says. Vaults’ proteins are highly conserved across the eukaryotes that have the particles, and analysis suggests the major vault protein (MVP), which makes up the bulk of the particles, was present in the last eukaryotic common ancestor.
Vaults remained undiscovered for so long because they are difficult to stain and difficult to differentiate from other cellular bodies such as glycogen particles. “Vaults are only 4% RNA so they don’t pick up stain like ribosomes do,” says Rome. “Under an electron microscope they would be just another grey blob. They had to be purified by accident in order to see them, and we were just lucky enough to have that accident.”
We now know that vaults are large, abundant and highly conserved – all traits that suggest an important cellular function. But almost 40 years since their discovery, neither Rome, nor anyone else, has figured out what that might be.
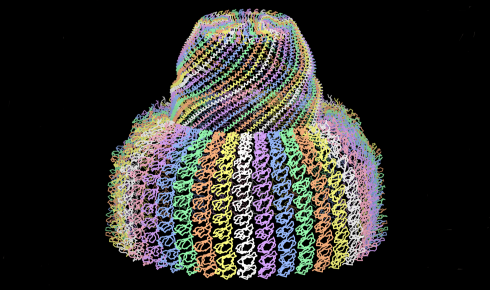
Finding a function
Rome and fellow UCLA researcher Dr Valerie Kickhoefer have spent decades trying to understand what purpose vaults might serve in cells. Rome is asked the question so often that his stock response is to joke that their function is “to help me put my three kids through college”. But the truth is, all manner of studies and experiments to try to deduce the function of vaults have failed to reach a conclusive answer.
Vaults are made up of three proteins and a small untranslated RNA. The MVP protein makes up the outer casing while the two other proteins are involved with packaging and stabilising the RNA cargo. Some organisms with MVP protein knocked out were found to be slightly ‘slow growers’, but MVP knockout mice were perfectly healthy. Losing the internal TEP1 protein, known to be a telomerase-RNA binding protein, also had little effect, with no noticeable effect on the telomeres of the mice, their ageing, or their health generally¹. Knocking out another vault protein, known as VPARP, led to mice with very slightly raised tumour growth when tumours were induced. Knocking out all three vault proteins, creating completely vaultless mice, also led to phenotypically normal mice, albeit with some subtle immune system issues².
None of the effects were strong – the vaultless organisms seemed “pretty much fine”, says Rome.
Promising leads have come and gone over the years, linking vaults with resistance to infections³, cell signalling⁴ and autophagy⁵. The over-expression of the major vault protein in certain lines of drug-resistant cancers led to vaults being a topic of interest in many studies of multidrug resistance⁶. Rome once thought vaults might be involved in transporting mRNA across the cell, because of the way they associate with the actin filaments running through cytoplasm, but this turned out to be a ‘rabbit hole’ that led nowhere.
While there is now decent evidence that vaults indirectly influence the immune system response and tumour development, there are still no strong clues as to a specific function. Rome has more ideas that he’d like to explore further. One hypothesis is that vaults, given their proteinous mass, could be a kind of bulk storage molecule for amino acids, akin to how glycogen particles store glucose.
Rome leans towards the idea that vaults are helping coordinate something in the cell cytoplasm – such as the various transient membrane-less cell bodies (ie stress granules and processing bodies) that have been found to have roles in the response to stresses like viral infection and cancer. “It’s very possible that they are moving things around the cell, but there’s no data. We just don’t know,” he says.
When Rome and his students tried to create recombinant vaults by splicing the major vault protein (MVP) gene into insects, they found something particularly interesting. The insects began producing vaults, but at no point could any free MVP be detected in the cell.
It turned out that the MVP was assembling into the characteristic barrel-shaped particle as the protein was being produced by the ribosome, or more accurately as multiple ribosomes travelled along the mRNA chain⁹.
As one MVP dimer is produced by one ribosome, it folds back on itself and connects with the next MVP dimer being produced by the ribosome moving along the mRNA behind it (see Figure 1, step 1, above). This continues as more and more ribosomes progress along the mRNA, with the dimers attaching to each other in a way that gradually forms a curved wall, like the staves of a barrel self-assembling.
When 39 MVP dimers come together and the first and last ones close the structure, the whole thing is pinched off and released into the cell.
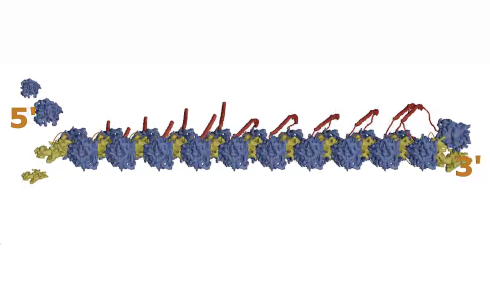
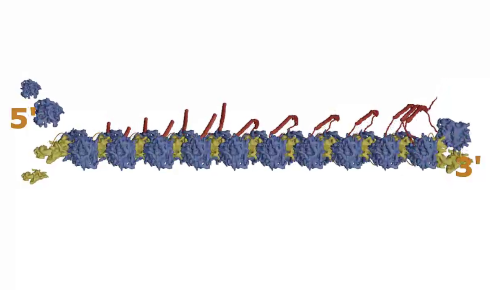
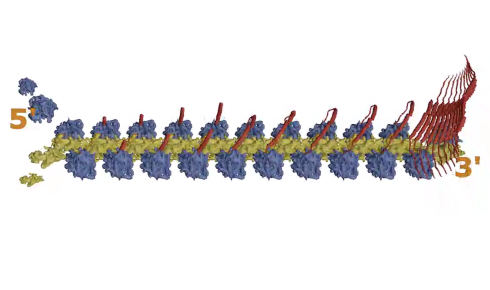
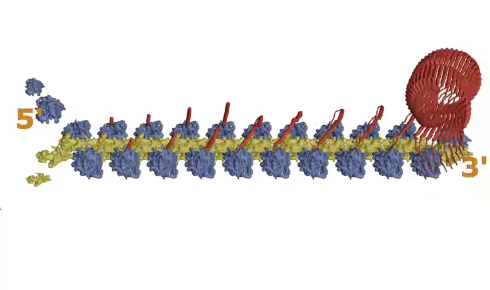
On to applications
Although the US’s National Institutes of Health funded Rome’s vault studies for 15 years, they eventually lost interest and the funding stopped. He says that starting with the particle and trying to find its function has been “exponentially more difficult” than starting with a function and trying to deduce a mechanism, “especially when you don’t have major eukaryotic knockout species like yeast, worms or flies available”.
Without funding to continue to investigate function, Rome has turned to exploring potential applications of vault particles. He and colleagues are working on an engineered anti-cancer vault that displays the compound CCL21 – a chemokine that normally acts in the lymphatic system, attracting dendritic cells and T-cells and stimulating them to attack tumour cells⁷. An advantage of using vault-based agents in immunotherapy is that they would negate the need for virus-transfected patient cells, and vaults are identical between people, so such nanomedicines could be used off the shelf. Vault Pharma, founded in 2013, plans to begin clinical trials for its engineered vault-based therapeutic for lung cancer next year and is expanding trials to treat other types of cancers. Other potential ideas being explored are vault-based pathogen or cancer vaccines.
In a completely different application of vaults, Rome’s colleague at UCLA Shaily Mahendra is exploring the use of vault nanoparticles packaged with enzymes to degrade environmental contaminants. Multiple enzymes for degrading organic pollutants, including manganese peroxidase, were encapsulated in vaults, which appeared to improve the stability of the enzymes without compromising their catalytic activity⁸.
Despite these interesting applications, still remarkably few scientists study vaults. Rome is aware of just a few other groups beyond his team and collaborators that have been exploring vault structure and engineering. “Nobody studies vaults. We’re basically it,” he says.
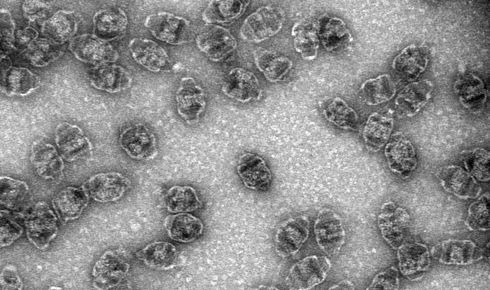
Solo study
Rome is now semi-retired, running a small lab, forging new collaborations, and producing humorous YouTube videos to spread the word about vaults and cell biology. He is hopeful that the function of the vault will be understood soon, but that requires more life science students to actually know they exist.
“The major cell biology textbooks describe all the major components of the human cell, but they never put a vault in there. OK, that’s because we don’t know what they do. But how many other things are out there that the textbooks ignore because we don’t know what they do? Vaults are such a big elephant in the room. But the pictures of the room leave the elephant out.”
Despite his long and seemingly lonesome quest to unlock the mystery of the vault, Rome says he is having more fun than ever with his research.
“People are contacting me, I’m finding some more labs that are interested in working on vaults. And I’ll be very happy if somebody figures out what vaults do before I die.”
Leonard H Rome is distinguished professor of biological chemistry at the David Geffen School of Medicine at UCLA and chief scientific officer of Vault Pharma.
Leonard Rome's public outreach videos on vaults can be found on YouTube.
vaultpharma.com
References
1) Liu, Y. et al. Vault poly(adp-ribose) polymerase is associated with mammalian telomerase and is dispensable for telomerase function and vault structure in vivo. Mol. Cell Biol. 24(12),
5314–5323 (2004).
2) Kickhoefer, V. A. & Rome, L. (unpublished).
3) Kowalski, M. P. et al. Host resistance to lung infection mediated by major vault protein in epithelial cells. Science 317(5834), 130–2 (2007).
4) Berger, W. et al. Vaults and the major vault protein: novel roles in signal pathway regulation and immunity. Cell. Mol. Life Sci. 66(1) 43–61 (2009).
5) Horos, R. et al. Vault RNA emerges as a regulator of selective autophagy. Autophagy 15(8), 1463–1464 (2019).
6) Kickhoefer, V. A. et al. Vaults are up-regulated in multidrug-resistant cancer cell lines. J. Biol. Chem. 273(15), 8971–8974 (1998).
7) Kar, U. K. et al. Novel CCL21-vault nanocapsule intratumoral delivery inhibits lung cancer growth. PLOS ONE 6(5), e18758 (2011).
8) Wang, M. et al. Vault nanoparticles packaged with enzymes as an efficient pollutant biodegradation technology. ACS Nano 9(11), 10931–10940 (2015).
9) Mrazek, J. et al. Polyribosomes are molecular 3D nanoprinters that orchestrate the assembly of vault particles. ACS Nano 8(11), 11552–11559 (2014).