The sense behind senescence
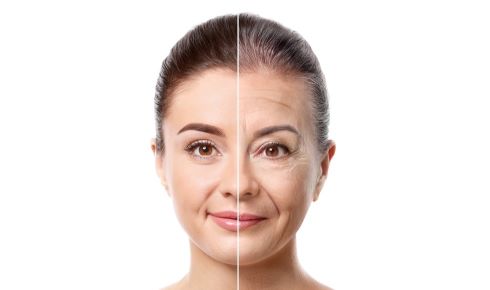
December 11th 2023
Anti-ageing is big business, but why do we age in the first place? Dr Andy Dobson explains the evolutionary logic of age-related deterioration
Will science ever defeat ageing? It’s a contentious question. It’s also a potentially lucrative one if you can answer in the affirmative – and a number of generously funded startups (Silicon Valley’s Altos Labs the most prominent among them) are attempting to do just that. For those of us on the sidelines, however, restricted by money and expertise to purely philosophical musings, a more rewarding enterprise might be to wonder why we need to do this in the first place. Why do we age?
It isn’t at all obvious when you stop and think about it. Natural selection would appear to be allowing the abandonment of a hard-won body – one that has survived predation, competition and all manner of other hardships, that has proved its worth as a reproductive vessel, that has done all that its genetic instructions have asked of it – and leaving it to slowly fall apart. Ageing only seems reasonable to us because we are so familiar with it, but for students of evolutionary biology it’s a question that demands a proper answer.
A good starting point is the insight of British biologist Sir Peter Medawar, who observed in 1952¹ that “the force of natural selection weakens with increasing age”. To explain this we must first clarify that the strength of selection pressures on a given trait is proportional to the cost of lacking it. Minor inconveniences might not be ‘seen’ by natural selection, whereas the inability to perform a basic function such as feeding will be harshly filtered out.
What would happen, though, if a trait showed itself only after an individual had already reared some offspring? Some of its fitness would be ‘in the bank’, so to speak, and hence couldn’t be lost. The trait would necessarily be less costly and would therefore prompt a correspondingly milder selection pressure. And, again, imagine that the trait appeared even later, when the individual had reared all of the offspring that it was ever going to have in its lifetime (and recognising that even a potentially ageless animal still has a limited lifespan, given the chances of predation or accident, and hence an upper limit on lifetime fitness). How costly is the trait now? All of that fitness has been accrued; it hardly matters at all if the individual were to drop dead. Put simply, the cost of death – and hence the selection pressure on avoiding it – declines over time.
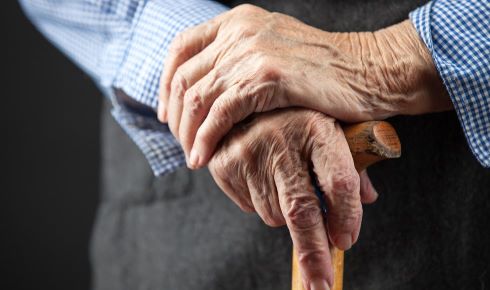
Medawar followed his observation with the hypothesis that a reduction over time in selection pressure would mean that a species could accumulate harmful genetic traits that only manifested themselves late in an individual’s lifetime. Those late-acting but harmful traits could collectively drive the progress of ageing.
This is an attractive idea but not quite complete, since if all other things were equal, then those late-acting traits would still be at least slightly disadvantageous, and lineages that held them would still eventually be outcompeted by those that didn’t.
The pleiotropy phenomenon
Enter, stage-left, antagonistic pleiotropy. Pleiotropy is the name given to the phenomenon whereby one gene (or distinct combination of genes) is associated with two or more unrelated physical characteristics – that is, has several distinct effects – and it gains the label ‘antagonistic’ if one effect is positive while another is negative. Crucially, if the positive effect occurs only in young, reproductive individuals, and the negative effect only in older ones, then the gene could be selected even though it essentially promotes ageing, due to that reduction in selection pressure over time.
Note that this is distinct from Medawar’s accumulation hypothesis, which simply says that harmful traits occurring later in life can end up in the genome because they aren’t as important as ones that occur early. Antagonistic pleiotropy is different – the late ‘bad’ trait will be actively favoured if accompanied by a ‘good’ early one that outweighs its effects.
Real-life data to support this hypothesis were scarce when American biologist George Williams first developed it in 1957, but things have changed since then. One of the best-documented examples comes from the ubiquitous laboratory nematode Caenorhabditis elegans, which is sufficiently simple that we can study the impacts of genetic changes with a high degree of precision.
In 1993 Cynthia Kenyon and colleagues at the University of California in San Francisco found that individuals with a mutation in the daf-2 gene experience a dramatic extension in lifespan², typically living more than twice as long as their ‘wild-type’ equivalents (not least due to the mutants’ enhanced resistance to pathogens). However, a slew of later work from this lab and others around the world has now demonstrated that daf-2 mutants pay for their longevity with a lowered rate of reproduction³. This reduction is only in the order of 18–20% but it takes effect when the worms are young and selection is strong, so when placed in direct competition with their wild-type brethren the daf-2 mutants are quickly outmatched.
This neat set of experimental evidence exemplifies antagonistic pleiotropy (the daf-2 gene mutation has several apparently unrelated effects) as well as Medawar’s insight into falling selection pressure – the doubled lifespan ought to more than compensate for the one-fifth reduction in reproduction, but the former happens earlier in life, thereby magnifying its impact.
Reproduction versus maintenance
An alternative hypothesis to account for the evolution of ageing starts with the premise that while there’s no particular reason why a body couldn’t be made to last indefinitely, it is nonetheless costly to do so. This is the ‘disposable soma’ theory, which follows this basic chain of reasoning: that reproduction is vital for fitness, and reproduction is energetically costly, and so energy used in reproduction cannot therefore be used for somatic (i.e. bodily) maintenance. Given that energy is limited by resource availability, somatic degradation is inevitable if you want to reproduce.
Disposable soma theory seems intuitively to account for the difference in life history strategies between species such as the field vole and the white rhinoceros. Field voles live for about a year, and females will have several litters of young during that time; female white rhinos, by contrast, may live for 50 years, and take six or seven years to mature, having a single calf every two or three years thereafter. Voles and rhinos occupy extremes of a rough continuum: breed fast and die young, or breed slow and die old. The continuum could also be seen in terms of other trade-offs, such as ‘invest in breeding and hope to reproduce before you are eaten or die from disease’ or ‘invest in defence against predators and pathogens so that you live long enough to breed’. All other things being equal, the average female vole and the average female rhino will come to be replaced by two voles and two rhinos, respectively, meaning that neither strategy is consistently more successful than the other.
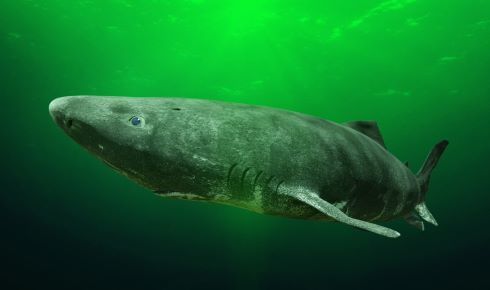
Determining whether the relative patterns of ageing in voles and rhinos are actually best explained by disposable soma theory or by antagonistic pleiotropy is not straightforward. Either (or both) could be playing the major role. Are voles in some sense ‘deciding’ to live fast and die young, or are their genomes so full of harmful late-acting genes that they have no choice but to reproduce as quickly as possible lest they die before they can have all their pups?
In order to identify disposable soma-type strategising in nature we need a species that has individuals that can display variation in the rate of ageing in response to external circumstances. Happily, honey bees fit the bill.
A female honey bee will either grow into a worker, with a lifespan measured in weeks or months, or a queen, which in some cases can live almost a decade. These are averages, however, and as such might not reflect varying rates of ageing (and therefore differing lifespan potential) but instead could be due to the relative hazards of their daily lives. Workers, after all, work hard, and often outside, where they are exposed to predators, extremes of weather and other natural dangers. Queens, on the other hand, may stay inside the hive their whole lives, fed and defended by thousands of their daughters.
More potentially revealing is the observation of consistent differences in average lifespan between workers who emerge at different times of year. Roughly speaking, summer workers live for about a month, busying themselves collecting pollen, while winter workers (who emerge from pupae in the autumn) typically live four or five times longer, having an easier time spent mostly in the hive until they start foraging in the spring. It’s possible that summer workers divert maximal amounts of energy to daily activities that are particularly important at that time of year (foraging, tending larvae and so on) and invest far less on somatic maintenance (which would arguably be wasted, given how likely it is that the worker would be killed before it could reach old age anyway). By contrast winter workers, whose duties are less onerous and lives less dangerous, might allocate energy differently, retaining some for themselves, thus allowing them to stay alive until the spring.
If that were the case, it would be an example of ‘plastic senescence’ – that is, a lack of predetermination in the rate of ageing and instead an ability to choose an optimal ageing strategy for different environments. Disposable soma theory would be vindicated. It’s a big ‘if’, of course, because – just as for queens versus workers – these average differences in lifespan could merely result from the differing hazards experienced by each cohort and not from altered rates of ageing. (The oft-reported six-week life expectancy of British junior officers on the Western Front during World War I had nothing to do with a strategic diversion of energy away from somatic maintenance, either.)
Aware of these conflicting explanations, Jack da Silva from the University of Adelaide, Australia, analysed detailed life history tables from several published studies that included data from hundreds of individually marked bees and used it to calculate the extrinsic mortality rates (deaths due to environmental hazards only) for separate cohorts of honey bee workers. Armed with these rates da Silva was able to use mathematical models to demonstrate that the difference in survival between cohorts was only partly due to external factors and partly due to intrinsic differences in the rates of ageing. In other words, honey bees were adjusting their bodily deterioration in order to allocate energy across their lifetimes in the most useful way possible given their time of emergence4.
Evolving understanding
We are neither bees nor nematode worms, but whether it is antagonistic pleiotropy or disposable soma theory that best explains the spidery march of wrinkles across our faces, one thing is certain: the technological war against our mortality will continue. The traditional weapons of medicine, agriculture and public health still win us new ground, and the so-called ‘cellular rejuvenation’ techniques of Altos Labs and their competitors may herald a great step forward.
We must be mindful, however, of the consequences. The perennial problem of balancing the needs of the retired population with the productivity of the workers will surely get worse; it will also become more unbalanced as the benefits of the technology accrue disproportionately to the wealthy. If the drive towards ever greater longevity is to yield more good than harm, we must plan our societies and economies accordingly and acknowledge that on a planet of finite resources, the gains of one generation are often the losses of the next. Natural selection is blind but we don’t have to be.
Andy Dobson is a research Fellow at the University of Stirling. His book, Flaws of Nature: The Limits & Liabilities of Natural Selection, is published by The History Press.
1) Medawar, P. B. An Unsolved Problem of Biology: An Inaugural Lecture Delivered at University College London. H. K. Lewis and Company (1952).
2) Kenyon, C. et al. A C. elegans mutant that lives twice as long as wild type. Nature 366(6454), 461–464 (1993).
3) Tissenbaum, H. A. & Ruvkun, G. An insulin-like signalling pathway affects both longevity and reproduction in Caenorhabditis elegans. Genetics 148(2), 703–717 (1998).
4) da Silva, J. Plastic senescence in the honey bee and the disposable soma theory. Am. Nat. 194(3), 367–380 (2019).